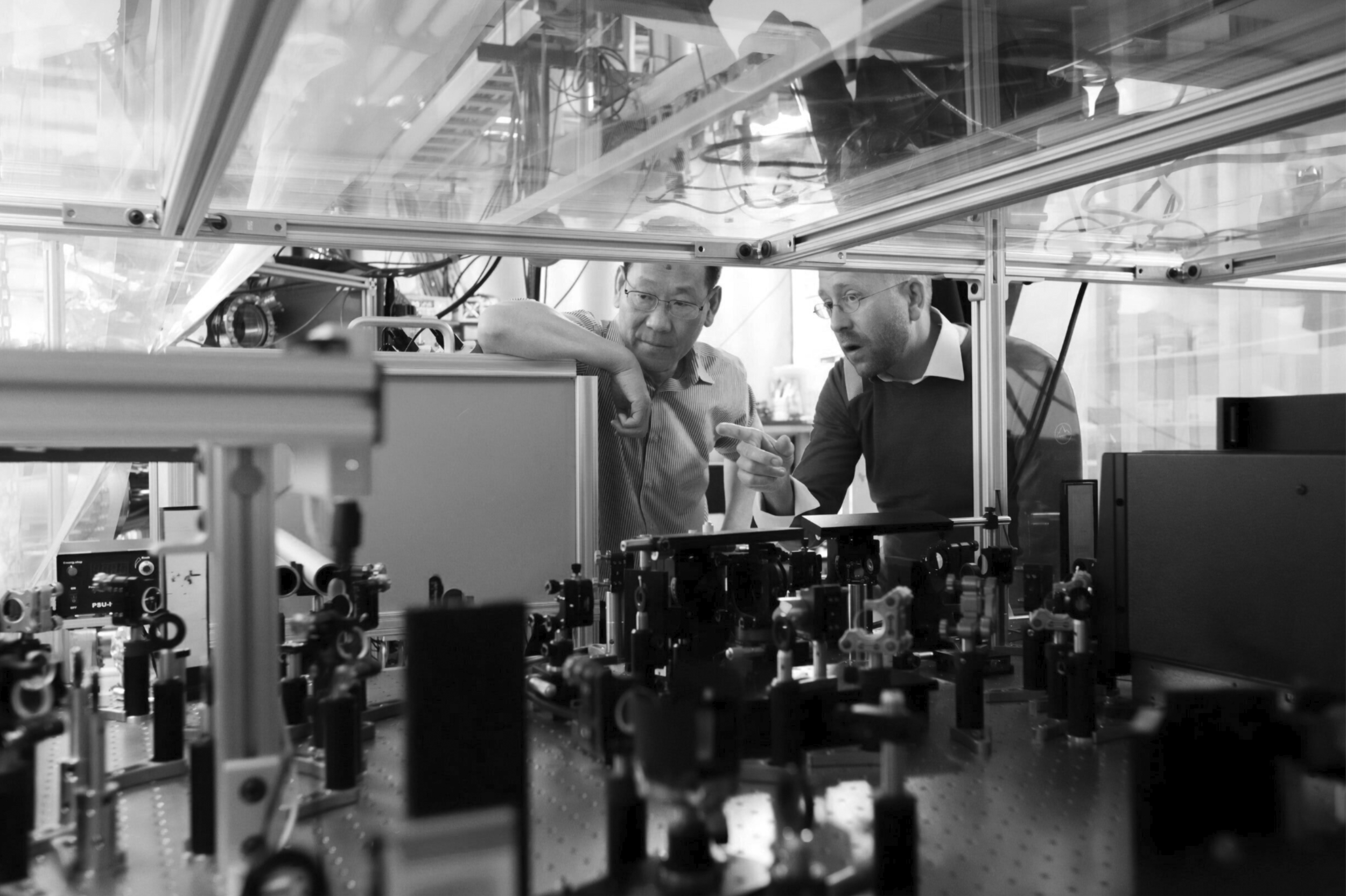
A STRATEGIC MANUFACTURING PARTNER FOR GLOBAL BRANDS
Powering over 3 billion devices across the globe
Your trusted power products partner
With nearly 50 years of experience, Salom is a leading global manufacturer of power supplies for consumer, medical, telecommunications and enterprise electronics products.
Original design & manufacturing
A trusted manufacturing partner to many Fortune 100 Companies.
Salom is at the forefront of power supply design and manufacture and for more than 40 years has maintained long term valued customer relationships. Working with new start-ups to globally recognised and respected brands, we are one of the leading power supply manufacturers world-wide.
License & distribution services
Licensing and Distribution services that complement our OEM business.
We complement your brand value by licensing applied to our area of expertise. We can then distribute the products through our established global channels alongside yours if appropriate. A 'win-win' often generating untapped potential returns from your brand under a clear licensing agreement while you concentrate on your core business.
Our power product partners
Salom products are trusted by some of the most successful electronics companies in the world, including…
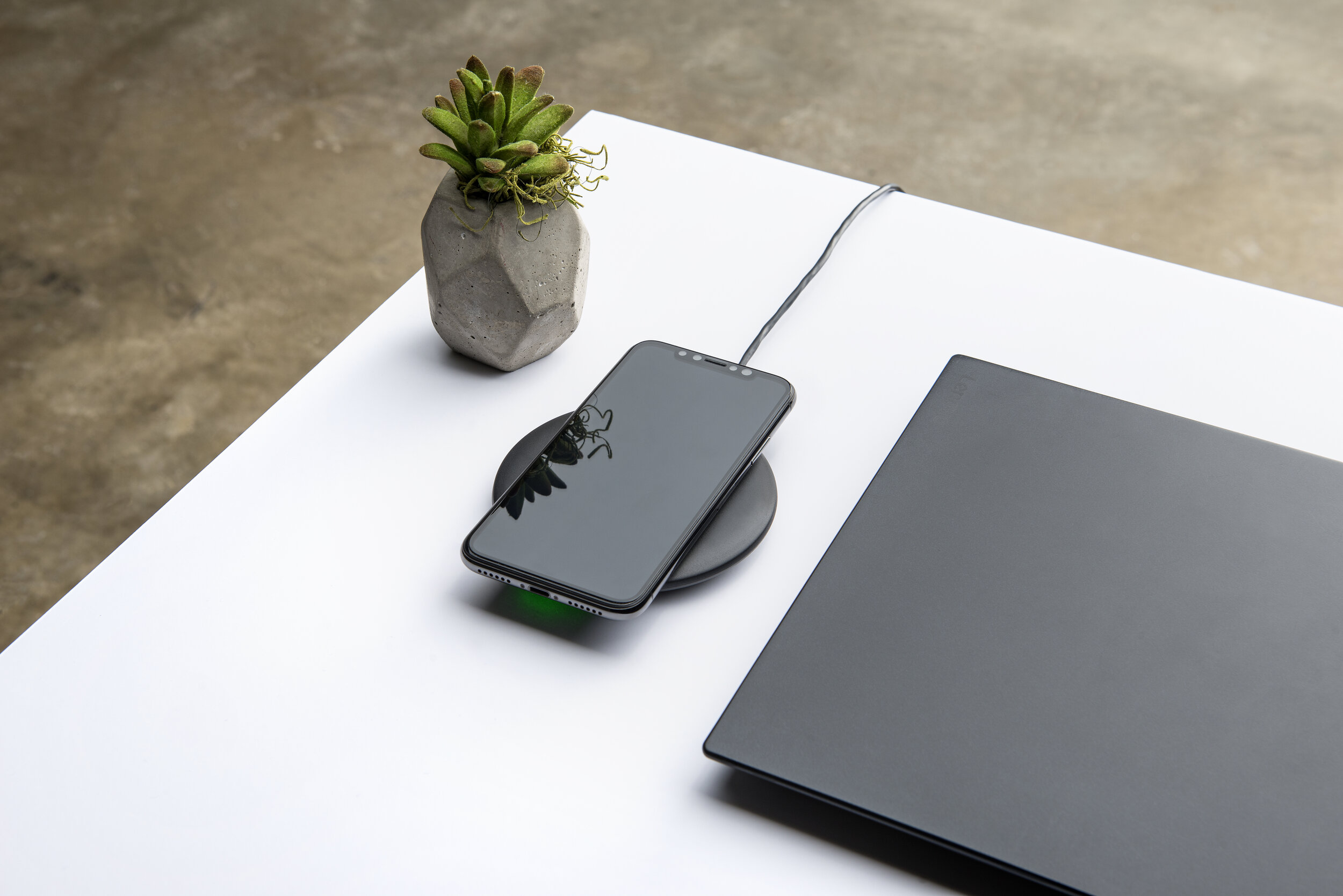
We are committed to providing outstanding power products and services of the highest quality
End-to-end turnkey capabilities
Engage
It all starts with an idea. We're happy to discuss early-stage concepts and provide valued industry recommendations on how to proceed with your project.
Design and develop
Once we have a specification, we will design and develop your product, ensuring it meets your requirements at every stage.
Certify and manufacture
We work with global 3P Safety Agencies to ensure all our products meet global legislation and can be manufactured at our approved global sites.
Maintain
Quality comes as standard. We maintain and report on production in accordance with agreed QA processes and legislative requirements.
News
Sustainability is a critical part of our value proposition and an area for continuous investment.
We look forwards to ongoing close collaboration with all our customers for the benefit of all.